The number of introduced forest pathogens are increasing – creating a crisis that is recognized by more scientists. These experts say tree diseases are reshaping both native and planted forests around the globe. The diseases are threatening biodiversity, ecosystem services, provision of products, and related human wellbeing. Some suggest that bioinvasions might threaten forests as much as climate change, while also undermining forests’ role in carbon sequestration.
Unfortunately, I see little willingness within the plant health regulatory community to tackle improving programs to slow introductions. Even when the scientists documenting the damage work for the U.S. Department of Agriculture – usually the U.S. Forest Service — USDA policy-makers don’t act on their findings. [I tried to spur a conversation with USDA 2 years ago. So far, no response.]
What the scientists say about these pests’ impacts
Andrew Gougherty (2023) – one of the researchers employed by the USDA Forest Service – says that emerging infectious tree diseases are reshaping forests around the globe. Furthermore, new diseases are likely to continue appearing in the future and threaten native and planted forests worldwide. [Full references are provided at the end of the blog.] Haoran Wu (2023/24) – a Master’s Degree student at Oxford University – agrees that arrival of previously unknown pathogens are likely to alter the structure and composition of forests worldwide. Weed, Ayers, and Hicke (2013) [academics] note that forest pests — native and introduced — are the dominant sources of disturbance to North American forests. They suggest that, globally, bioinvasions might be at least as important as climate change as threats to the sustainability of forest ecosystems. They are concerned that recurrent forest disturbances caused by pests might counteract carbon mitigation strategies.
Scientists have proclaimed these warnings for years. Five years ago, Fei et al. (2019) reported that the 15 most damaging pests introduced to the United States — cumulatively — had already caused tree mortality to exceed background levels by 5.53 teragrams of carbon per year. As these 15 pests spread and invasions intensify, they threaten 41.1% of the total live forest biomass in the 48 coterminous states. Poland et al. (2019) (again – written by USFS employees) document the damage to America’s forest ecosystems caused by the full range of invasive species, terrestrial and aquatic.
Fei et al. and Weed, Ayers, and Hicke (2013) also support the finding that old, large trees are the most important trees with regard to carbon storage. This understanding leads them to conclude that the most damaging non-native pests are the emerald ash borer, Dutch elm disease fungi, beech bark disease, and hemlock woolly adelgid. As I pointed out in earlier blogs, other large trees, e.g., American chestnut and several of the white pines, were virtually eliminated from much of their historical ranges by non-native pathogens decades ago. These same large, old, trees also maintain important aspects of biological diversity.
It is true that not all tree species are killed by any particular pest. Some tree genera or species decrease while others thrive, thus altering the species composition of the affected stands (Weed, Ayers, and Hicke). This mode of protection is being undermined by the proliferation of insects and pathogens that cumulatively attack ever more tree taxa. And while it is true that some of the carbon storage capacity lost to pest attack will be restored by compensatory growth in unaffected trees, this faster growth is delayed by as much as two or more decades after pest invasions begin (Fei et al.).
Still, despite the rapid rise of destructive tree pests and disease outbreaks, scientists cannot yet resolve critical aspects of pathogens’ ecological impacts or relationship to climate change. Gougherty notes that numerous tree diseases have been linked to climate change or are predicted to be impacted by future changes in the climate. However, various studies’ findings on the effects of changes in moisture and precipitation are contradictory. Wu reports that his study of ash decline in a forest in Oxfordshire found that climate change will have a very small positive impact on disease severity through increased pathogen virulence. Weed, Ayers, and Hicke go farther, making the general statement that despite scientists’ broad knowledge of climate effects on insect and pathogen demography, they still lack the capacity to predict pest outbreaks under climate change. As a result, responses intended to maintain ecosystem productivity under changing climates are plagued by uncertainty.
Clarifying how disease systems are likely to interact with predicted changes in specific characteristics of climate is important — because maintaining carbon storage levels is important. Quirion et al. (2021) estimate that, nation-wide, native and non-native pests have decreased carbon sequestration by live forest trees by at least 12.83 teragrams carbon per year. This equals approximately 9% of the contiguous states’ total annual forest carbon sequestration and is equivalent to the CO2 emissions from more than 10 million passenger vehicles driven for one year. Continuing introductions of new pests, along with worsening effects of native pests associated with climate change, could cause about 30% less carbon sequestration in living trees. These impacts — combined with more frequent and severe fires and other forest disturbances — are likely to negate any efforts to improve forests’ capacity for storing carbon.
Understanding pathogens’ interaction with their hosts is intrinsically complicated. There are multiple biological and environmental factors. What’s more, each taxon adapts individually to the several environmental factors. Wu says there is no general agreement on the relative importance of the various environmental factors. The fact that most forest diseases are not detected until years after their introduction also complicates efforts to understand factors affecting infection and colonization.
The fungal-caused ash decline in Europe is a particularly alarming example of the possible extent of such delays. According to Wu, when the disease was first detected – in Poland in 1992 – it had already been present perhaps 30 years, since the 1960s. Even then, the causal agent was not isolated until 2006 – or about 40 years after introduction. The disease had already spread through about half the European continent before plant health officials could even name the organism. The pathogen’s arrival in the United Kingdom was not detected until perhaps five years after its introduction – despite the country possessing some of the world’s premier forest pathologists who by then (2012) knew what they to look for.
Clearly, improving scientific understanding of forest pathogens will be difficult. In addition, effective policy depends on understanding the social and economic drivers of trade, development, and political decisions are primary drivers of the movement of pathogens. Wu calls for collaboration of ecologists, geneticists, earth scientists, and social scientists to understand the complexity of the host-pathogen-surrounding system. Bringing about this new way of working and obtaining needed resources will take time – time that forests cannot afford.
However, Earth’s forests are under severe threat now. Preventing their collapse depends on plant health officials integrating recognition of these difficulties into their policy formulation. It is time to be realistic: develop and implement policies that reflect the true level of threat and limits of current science.
Background: Rising Numbers of Introductions
Gougherty’s analysis of rising detections of emerging tree diseases found little evidence of saturation globally – in accord with the findings of Seebens et al. (2017) regarding all taxa. Relying on data for 24 tree genera, nearly all native to the Northern Hemisphere, Gougherty found that the number of new pests attacking these tree genera are doubling on average every 11.2 years. Disease accumulation is increasing rapidly in both regions where hosts are native and where they are introduced, but more rapidly in trees’ native ranges.This finding is consistent with most new diseases arise from introductions of pathogens to naïve hosts.
Gougherty says his estimates are almost certainly underestimates for a number of reasons. Countries differ in scientific resources and their scientists’ facility with English. Scientists are more likely to notice and report high-impact pathogens and those in high-visibility locations. Where national borders are closer, e.g., in Europe, a minor pest expansion can be reported as “new” in several countries. New pathogens in North America appear to occur more slowly, possibly because the United States and Canada are very large. He suggests that another possible factor is the U.S. (I would add Canada) have adopted pest-prevention regulations that might be more effective than those in place in other regions. (See my blogs and the Fading Forest reports linked to below for my view of these measures’ effectiveness.)
Wu notes that reports of tree pathogens in Europe began rising suddenly after the 1980s. He cites the findings by Santini et al. (2012) that not only were twice as many pathogens detected in the period after 1950 than in the previous 40 years, the region of origin also changed. During the earlier period, two-thirds of the introduced pathogens came from temperate North America. After 1950, about one-third of previously unknown disease agents were from temperate North America. Another one-third was from Asia. By 2012, more than half of plant infectious diseases were caused by introduction of previously unknown pathogens.
What is to be done?
Most emerging disease agents do not have the same dramatic effects as chestnut blight in North America, ash dieback in Europe, or Jarrah dieback in Australia. Nevertheless, as Gougherty notes, their continued emergence in naïve biomes increases the likelihood of especially damaging diseases emerging and changing forest community composition.
Gougherty calls for policies intended to address both the agents being introduced through trade, etc., and those that emerge from shifts in virulence or host range of native pathogens or changing environmental conditions. In his view, stronger phytosanitary programs are not sufficient.
Wu recommends enhanced monitoring of key patterns of biodiversity and ecosystem functioning, He says these studies should focus on the net outcome of complex interactions. Wu also calls for increasing understanding of key “spillover” effects – outcomes that cannot be currently assessed but might impact the predicted outcome. He lists several examples:
- the effects of drought–disease interactions on tree health in southern Europe,
- interaction between host density and pathogen virulence,
- reproductive performance of trees experiencing disease,
- effect of secondary infections,
- potential for pathogens to gain increased virulence through hybridization.
- potential for breeding resistant trees to create a population buffer for saving biological diversity. Wu says his study of ash decline in Oxfordshire demonstrates that maintaining a small proportion of resistant trees could help tree population recovery.
Quirion et al. provide separate recommendations with regard to native and introduced pests. To minimize damage from the former, they call for improved forest management – tailored to the target species and the environmental context. When confronting introduced pests, however, thinning is not effective. Instead, they recommend specific steps to minimize introductions via two principal pathways, wood packaging and imports of living plants. In addition, since even the most stringent prevention and enforcement will not eliminate all risk, Quirion et al. advocate increased funding for and research into improved strategies for inspection, early detection of new outbreaks, and strategic rapid response to newly detected incursions. Finally, to reduce impacts of established pests, they recommend providing increased and more stable funding for classical biocontrol, research into technologies such as sterile-insect release and gene drive, and host resistance breeding.
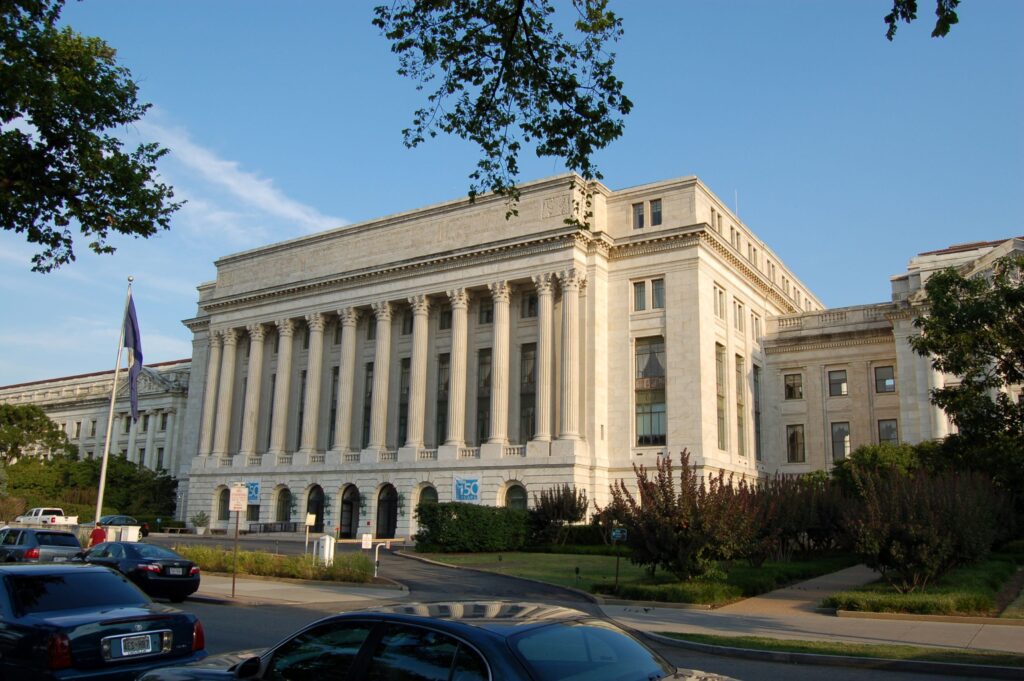
Remember: reducing forest pest impacts can simultaneously serve several goals—carbon sequestration, biodiversity conservation, and perpetuating the myriad economic and societal benefits of forests. See Poland et al. and the recent IUCN report on threatened tree species.
SOURCES
Barrett, T.M. and G.C. Robertson, Editors. 2021. Disturbance and Sustainability in Forests of the Western United States. USDA Forest Service Pacific Northwest Research Station. General Technical Report PNW-GTR-992. March 2021
Clark, P.W. and A.W. D’Amato. 2021. Long-term development of transition hardwood and Pinus strobus – Quercus mixedwood forests with implications for future adaptation and mitigation potential. Forest Ecology and Management 501 (2021) 119654
Fei, S., R.S. Morin, C.M. Oswalt, and A.M. 2019. Biomass losses resulting from insect and disease invasions in United States forests. Proceedings of the National Academy of Sciences. www.pnas.org/cgi/doi/10.1073/pnas.1820601116
Gougherty AV (2023) Emerging tree diseases are accumulating rapidly in the native and non-native ranges of Holarctic trees. NeoBiota 87: 143–160. https://doi.org/10.3897/neobiota.87.103525
Lovett, G.M., C.D. Canham, M.A. Arthur, K.C. Weathers, and R.D. Fitzhugh. 2006. Forest Ecosystem Responses to Exotic Pests and Pathogens in Eastern North America. BioScience Vol. 56 No. 5 May 2006
Lovett, G.M., M. Weiss, A.M. Liebhold, T.P. Holmes, B. Leung, K.F. Lambert, D.A. Orwig, F.T. Campbell, J. Rosenthal, D.G. MCCullough, R. Wildova, M.P. Ayres, C.D. Canham, D.R. Foster, S.L. Ladeau, and T. Weldy. 2016. Nonnative forest insects and pathogens in the United States: Impacts and policy options. Ecological Applications, 26(5), 2016, pp. 1437-1455
Poland, T.M., Patel-Weynand, T., Finch, D., Miniat, C. F., and Lopez, V. (Eds) (2019), Invasive Species in Forests and Grasslands of the United States: A Comprehensive Science Synthesis for the United States Forest Sector. Springer Verlag.
Quirion, B.R., G.M. Domke, B.F. Walters, G.M. Lovett, J.E. Fargione, L. Greenwood, K. Serbesoff-King, J.M. Randall, and S. Fei. 2021 Insect and Disease Disturbance Correlate With Reduced Carbon Sequestration in Forests of the Contiguous US. Front. For. Glob. Change 4:716582. [Volume 4 | Article 716582] doi: 10.3389/ffgc.2021.716582
Weed, A.S., M.P. Ayers, and J.A. Hicke. 2013. Consequences of climate change for biotic disturbances in North American forests. Ecological Monographs, 83(4), 2013, pp. 441–470
Wu, H. 2023/24. Modelling Tree Mortality Caused by Ash Dieback in a Changing World: A Complexity-based Approach MSc/MPhil Dissertation Submitted August 12, 2024. School of Geography and the Environment, Oxford University.
Posted by Faith Campbell
We welcome comments that supplement or correct factual information, suggest new approaches, or promote thoughtful consideration. We post comments that disagree with us — but not those we judge to be not civil or inflammatory.
For a detailed discussion of the policies and practices that have allowed these pests to enter and spread – and that do not promote effective restoration strategies – review the Fading Forests report at https://treeimprovement.tennessee.edu/
or