I have advocated for considerably expanding efforts to breed trees resistant to non-native pests (including pathogens) for a decade. Again and again, I and others have pointed out the dire consequences for our forests if we Americans do not rise to the challenge.
In 2014, Scott Schlarbaum – coauthor of Fading Forests III – American Forests: What Choice Will We Make? warned that without restoration becoming an integral part of a strategy addressing non-native plant pests, American ecosystems are doomed to continuing transformation. Once established, a non-native pest is never eliminated, but its impact can be reduced through a combination of measures – as long as support is made available. Scott advised initiating a germplasm conservation strategy when invasion is imminent or once the pest is likely to become a resident pest. (See Chapter 6).
I have posted seven blogs since August 2021 describing the current status of various efforts and urging the U.S. Government and conservation organizations to step up. [To view these blogs, go to www.nivemnic.us, scroll below Archives to “Categories” and click on “resistance breeding.”
More, and Recent, Voices: Implications of Not Acting
More recently, several USDA Forest Service (USFS) experts, including Richard Sniezko, C. Dana Nelson, and Jennifer Koch, have published articles making the same point. These scientists note that many of the decimated species were formerly among the most common trees in our forests. Therefore, the cumulative effect of their disappearance on forest species composition and function is multiplied.
One blog, posted in 2022, is particularly pertinent. It summarizes a special issue of the journal Plants, People, Planet devoted to resistance breeding. The opening essay, by R.J.A. Buggs, concisely reviews six major reasons why so many believe that resistance breeding is a failed strategy.
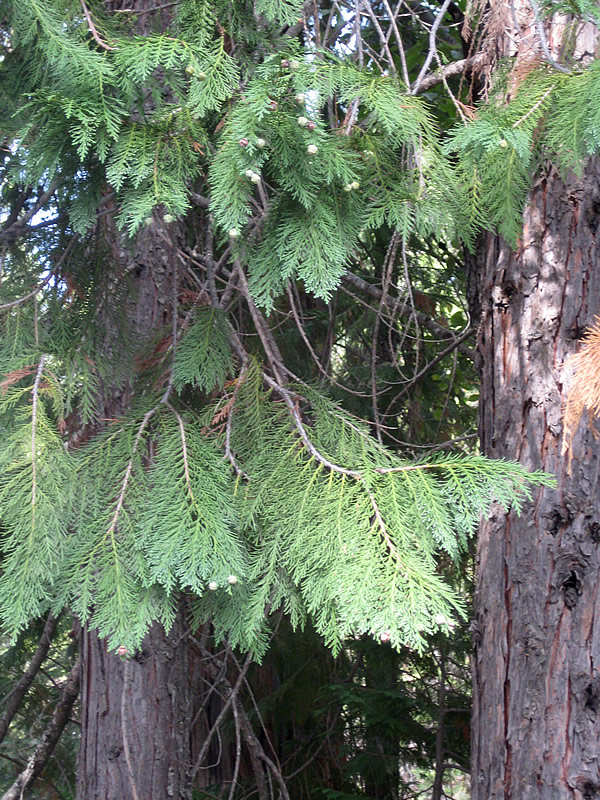
Others say there have been successes – all through application of classic tree improvement measures, not “genetic engineering.” Pike, Koch and Nelson (2021) list as successes Port-Orford-cedar (Chamaecyparis lawsoniana), the western five-needle pine species, koa (Acacia koa), and resistance to fusiform rust (Cronartium quercuum f. sp. fusiforme) in the commercially-important loblolly (Pinus taeda) and slash (P. elliottii) pines. They also cite encouraging progress by The American Chestnut Foundation (TACF) through backcross breeding of America and Asian chestnuts and a USFS/private foundation effort to expand the genetic base of American elms (Ulmus americana). I regret to say this, but some of these efforts seem to me to be still in experimental stages or — at best — early in widespread – ‘though still experimental — plantings.
Participants in a 2021 Purdue University workshop have again called for greatly expanding breeding. See the special issue of New Forests, Vol. 54 Issue 4. Once again, experts reiterate the urgency of acting, then outline the opportunities and challenges.
In one of the articles (Jacobs et al.) several people – including me! – note that several keystone tree species or genera in North America and Europe have been driven to functional extinction by non-native pests. By this we mean they are no longer sufficiently abundant and/or of adequate size to reproduce sexually or perform their ecological function. Examples include – on both continents – ashes (Fraxinus) and elms; and on North America – American chestnut (Castanea dentata), butternut (Juglans cinerea), and whitebark pine (Pinus albicaulis).If these threats are left unchecked, these at-risk tree species might develop truncated ranges, lose genetic diversity, and face becoming threatened, endangered, or extinct.
In another article, Nelson says the question that should be asked about applying genetic engineering (GE) techniques to tree breeding is whether we should let a species be reduced to a marginal role — or disappear — when GE provides a solution to saving and restoring the species. His case study is a detailed history of TACF’s development of a transgenic American chestnut (called “Darling 58”). He points out that decades of breeding efforts were based on the hope of developing blight resistance within the native gene pool or to obtain resistance from related species through hybridization. However, those efforts have not yet provided trees suitable for restoring the “king of the Appalachian forest” to native landscapes. Nelson wrote his description before TACF discovered flaws in the GE trees they had been working with and decided to pursue different GE “lines” (see below).
Barriers
The overall strategy is clear. Schlarbaum, Sniezko, and Dana Nelson all describe essentially the same steps, built on the same kinds of expertise and facilities.
Of course, each species will require years of input by a range of experts. These challenges are not trivial. However, the experts named above agree that the principal barrier is the absence of sustained, long-term commitment of resources and facilities. With sufficient resources, many of the scientific challenge can be overcome for at least some of the species at risk.
So, what are the scientific challenges? First, scientists must assess whether the tree species contains sufficient genetic variation in resistance. This involves locating candidate resistant trees; developing and applying short-term assay(s) to screen hundreds or thousands of candidate trees; and determining the levels of resistance present. Second, scientists must develop resistant planting stock for use in restoration. This stage includes scaling up the screening protocol; selecting the resistant candidates or progeny to be used; breeding to increase resistance; establishing seed orchards or other methods to deliver large numbers of resistant stock for planting; and additional field trials to further validate and delineate resistance. Sniezko and Koch (2017) and Sniezko and Nelson (2022) discuss the challenges and describe successes.
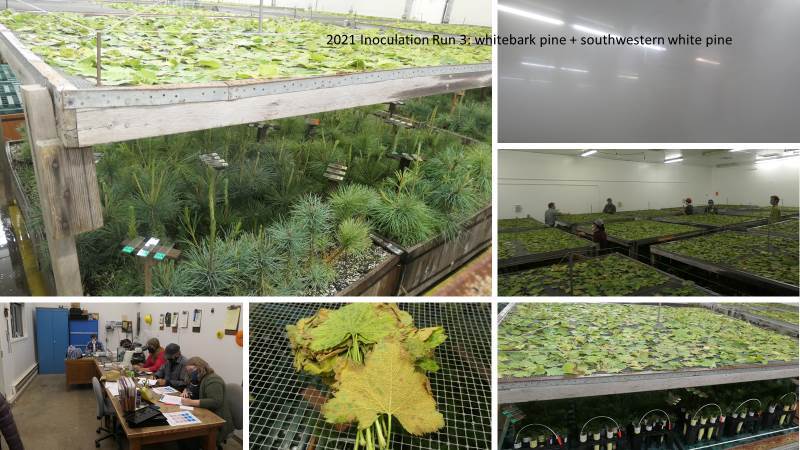
Complicating the restoration phase is the fact that the resistant tree must be able to thrive and compete in an ecosystem that has changed greatly from that in which it formerly resided. Causes of these changes include repercussions from the absence of the tree species – and possibly associated species; the possible presence of other biotic stresses (pests); and climate change. This is discussed by Nelson (2022). See also my blog.
Successfully completing these steps requires a long-term commitment, which includes significant funding and strong supportive infrastructure. Schlarbaum pointed out that the public and politicians don’t understand the complexity of the restoration challenge and the resources required. He documented the shrinking tree improvement infrastructure as of 2014. At that time, funding for all USFS regional breeding programs was just $6 million. State and land grant university breeding programs were fragmented and seriously underfunded. Only 28 states still had some type of tree improvement activity – and some of these programs were only seed orchards, not active breeding and testing programs. Members of university-industrial cooperatives focus on a small number of commercial species – which are not the species threatened by non-native pests. I believe these resources have shrunk even farther in the decade since 2014.
A separate source of funds for resistance breeding is the Forest Health Protection program, which is under the Deputy Chief for State, Private, and Tribal Forestry rather than the Deputy Chief for Research and Development. While nation-wide data on seed or scion collection or screening to identify and evaluate genetic resistance are poorly reported, Coleman et al. indicate that the USFS Dorena Genetic Resource Center screens unspecified “hundreds” of seed lots for resistance to pathogens annually. The Center also participates in seed, cone, and scion collections, especially of white pines vulnerable to white pine blister rust (WPBR). Supplemental Table S3 lists projects funded over the two decades analyzed by Coleman et al. (2011 – 2020). These included efforts to identify and evaluate possible genetic bases for resistance to, e.g., hemlock woolly adelgid, balsam woolly adelgid, laurel wilt, emerald ash borer, butternut canker, rapid ʻōhiʻa death; and gene conservation for eastern hemlock, ashes, chestnut, in addition to the five-needle pines. Currently, FHP allocates $1.2 million annually to support the group of activities called Genetic Conservation, Resistance and Restoration (R. Cooksey, pers. comm.).
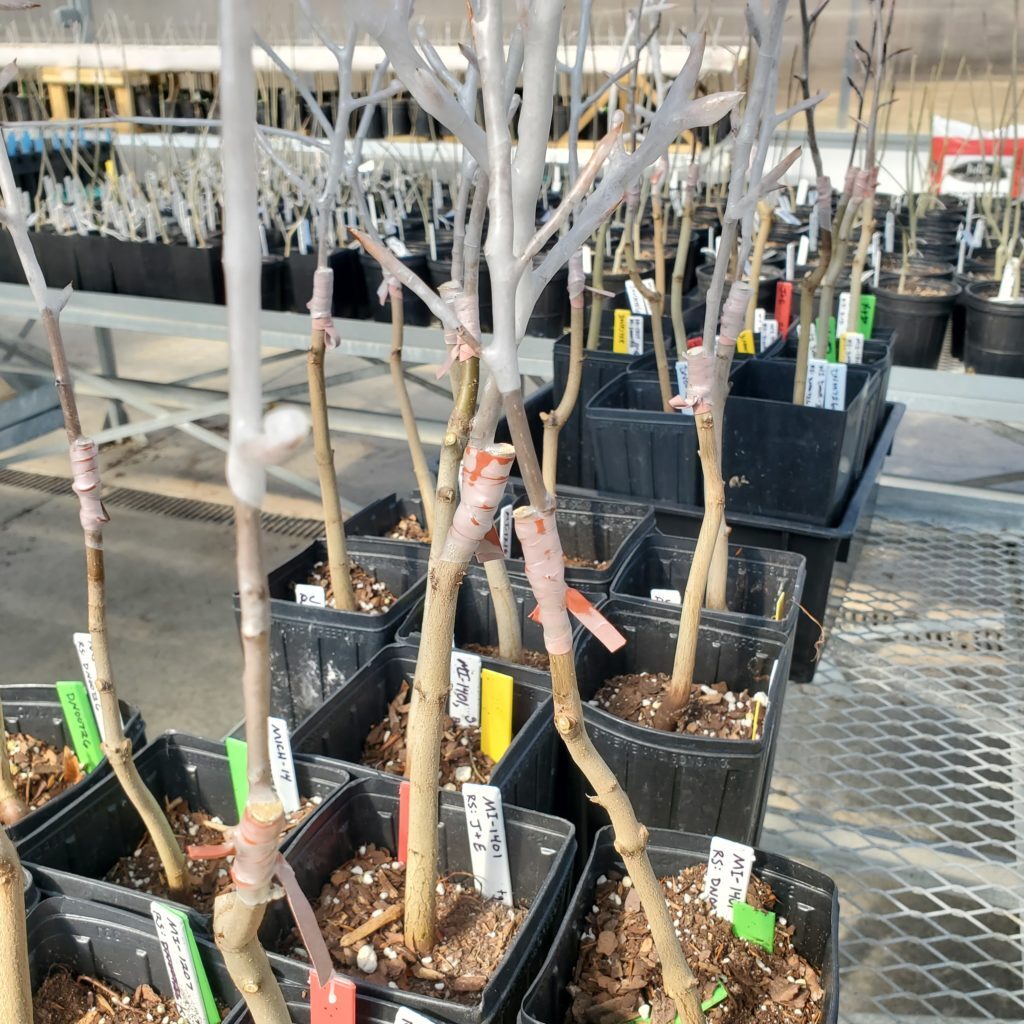
USFS scientists involved in these projects describe challenges arising from efforts to cobble together funding from these many sources to support coherent programs. Overall funding levels still fall short of the need, and failure to obtain funding for one component of a program stymies the entire endeavor.
However, some developments are encouraging. The number of private foundations devoted to tree breeding has increased in the last decade. The American Chestnut Foundation (TACF) and American Chestnut Cooperators Foundation (ACCF) have been joined by the White Pine Ecosystem Foundation, the Great Lakes Basin Forest Health Collaborative, Forest Restoration Alliance, ‘Ohi‘a Disease Resistance Program … These organizations raise awareness, coordinate efforts by multiple parties, and provide opportunities for individuals to contribute funds and volunteer work.
In Hawai`i, disease resistance programs with both koa (Dudley et al.) and ʻōhiʻa ((Metrosideros polymorpha) (Luiz et al.) are active. Work with ash species to find and develop resistance to emerald ash borer is under way but limited due to lack of funds.
Finally, we can persuade Congress to incorporate the provisions of two bills, H.R. 3174 and S. 1238, into the next Farm Bill. The bills would, inter alia, create two grant program. One would fund research addressing specific questions impeding the recovery of native tree species that have suffered severe levels of mortality caused by non-native plant pests. The second would fund implementation of projects to restore these pest-decimated tree species to the forest.
Funded projects would be required to be part of a forest restoration strategy that incorporates a majority of the following components:
(1) Collection and conservation of native tree genetic material;
(2) Production of propagules of the target tree species in numbers sufficient for landscape-scale restoration;
(3) Preparation of planting sites in the target tree species’ former habitats;
(4) Planting of native tree seedlings; and
(5) Post-planting maintenance of native trees.
For a detailed description, see this blog.
Details:
Facilities needed to support successful breeding programs
Sniezko and Nelson identified these needs as follows:
(a) growing space (e.g., greenhouses);
(b) seed handling and cold storage capacity;
(c) inoculation infrastructure;
(d) field sites for testing;
(e) database capability for collecting, maintaining, and analyzing data;
(f) areas for seed orchard development;
(g) skilled personnel (tree breeders, data managers, technicians, administrative support personnel, and access to expertise in pathology and entomology).
There are very few facilities dedicated primarily to development of populations of trees with resistance to non-native pests; the most notable is the Dorena Genetic Resource Center. Even the existing programs require significant funding increases to accelerate current programs or expand to address additional species. Sniezko and Nelson stress further that a resistance breeding program has different objectives, magnitude and focus than most research projects. It is applied science, that is, an action-oriented effort that is solution-minded—countering the impact of a major disturbance caused by a pest (in our case, a non-native pest).
Schlarbaum provides a shorter but similar list of facilities needed:
- production of propagules (seed or clones);
- mass propagation in growing facilities, e.g., bare-root seedling nursery or greenhouses;
- site preparation of former habitat and planting; and
- post-planting maintenance.
Schlarbaum emphasized that each of these activities requires different skill sets, equipment, facilities, and infrastructure.
Genetic Engineering as a Specific Tool
There is considerable interest in the potential role of genetic engineering in pest resistance breeding. None of the successful programs world-wide has yet used genetic engineering (Sniezko and Koch 2017). While incorporating it into holistic breeding programs might result in greater efficiency for certain processes, it raises legal and social acceptability issues. Jacobs et al. discuss the type of education and outreach program needed to generate widespread public support this approach to tree species “rescues.” They call for USDA Forest Service to lead this education effort.
The focus of the 2021 workshop hosted by Purdue University was to explore the pros and cons of using biotechnology in restoring pest-threatened forest tree species. The special issue of New Forests contains several participants’ analyses.
The overall conclusions are that:
- “Genetic engineering” – defined as “any technique that uses recombinant, synthesized, or amplified nucleic acids to modify a genome” – is only one type of biotechnology applicable to tree breeding. Other biotechnologies include tissue culture-based propagation, molecular-based genetic markers, gene cloning and sequencing, and genome mapping and sequencing.
- These new technologies can increase the efficiency of more traditional breeding techniques, However, biotechnologies cannot substitute for holistic programs that incorporate all helpful methods. Careful consideration goes into selecting which techniques are appropriate for a particular host-pest system.
- Each tree species has unique needs regarding seed or scion collection; seedling propagation in nurseries; site preparation and planting techniques; and management of regeneration after its re-introduction into forests. Scientists don’t yet understand these various needs of many threatened species.
- In the eastern U.S., the tree-breeding infrastructure is based in the Southeast and focused on a few pine species grown commercially. The facilities do not match the greatest need. That is, many of the at-risk species are hardwoods native to the Northeast.
- Current resources are inadequate to support the sustained, long-term commitment of resources and facilities necessary to be successful.
Dana Nelson addressed the role of genetic engineering (GE) in detail. He emphasized repeatedly that GE is not a short-cut to tree improvement. Incorporating a GE component does not avoid the other steps. It can, though, provide new possibilities to address problems. Nelson says the crucial, initial question is – can GE solve the specific forest conservation or management problem more effectively and efficiently than existing methods? There are some important subtleties to consider. First, success does not require achieving immunity (100% resistance); the level of resistance needs to be only sufficient to allow the tree species to survive, reproduce and co-evolve with the pest. Second, “efficiency” is an important consideration. We cannot afford delay because during those years or decades the wild tree loses genetic variability as more trees die. Also, changes in the environment continues to change, and the decimated tree species is not adapting.
If genetic engineering promises to contribute meaningfully, then the breeders must answer several follow-up questions before proceeding to develop a specific plan. Nelson also stresses that the planned activities must be integrated with an ongoing tree breeding program to ensure project success.
Nelson provides a lengthy description of the process of integrating genetic engineering into tree breeding programs.
GE in Chestnut Breeding – Setback
The most prominent breeding effort incorporating genetic engineering in the U.S. has been The American Chestnut Foundation’s (TACF) program to restore American chestnut (Castanea dentata). For decades, TACF has pursued development of trees resistant to the fungus which causes chestnut blight (Cryphonectria parasitica). Over the past decade, hopes have centered on a genetically engineered line into which was inserted a gene from wheat (oxalate oxidase; OxO). The OxO gene detoxifies the oxalic acid produced by the chestnut blight fungus and thus prevents the cankers from killing the tree.
Years of tests have shown the gene to be effective and to cause no environmental harm. In 2023, when trees in outside test plots grew larger, scientists observed disappointing results. Trees’ blight tolerance varied greatly. Worse, resistant trees grew more slowly and exhibited lower overall fitness. [For a full discussion of the issues, visit TACF’s website] Prompted by these disappointments, scientists carried out further molecular analyses. They found that the OxO gene was on a different chromosome than expected.
TACF researchers now suspect that the trees’ variable performance stems primarily from the placement of the OxO gene and the fact that the gene is always “switched on”. That constant expression appears to result in high metabolic costs for the trees. Since all the genetic lines developed to date have this defect, TACF is no longer pursuing research efforts with any of the GE trees developed to date. The Foundation believes it would be irresponsible to continue efforts – by itself and by partners – focused on a genetic line that looks unable to compete successfully when introduced to the forest.
Instead, TACF has begun investigating other transgenic lines that use a “wound inducible” promoter that switches on the OxO gene only in cells where the plant is wounded. Researchers at both the State University of New York College of Environmental Science and Forestry (SUNY-ESF) and the University of Georgia are working with a variety of inducible promoters. TACF is also testing whether inducible OxO expression can be “stacked”onto genes for blight resistance present in the backcross hybrids. Finally, TACF and Virginia Tech are also exploring whether resistance can be enhanced by insertion of genes from Chinese chestnut directly into American chestnut using methods similar to OxO insertion.
It will be years before we know if these approaches provide sufficient levels of resistance. TACF will undertake more extensive testing for efficacy through the tree’s full life cycle – in the lab, greenhouse, and field – before submitting a new GE organism to regulators for review. Meanwhile, it will continue rigorous testing for plant health and environmental risks and will strengthen the cooperative structure to facilitate sharing of intellectual property and provide full transparency.
The Darling GE line was the most important transgenic hybrid chestnut line TACF had invested in. So this is a major setback – and comes when regulatory approval seemed near.
Let’s keep this in perspective, however. As a colleague has said, based on his years of teaching science to middle school students, “There are no failures in science, just reductions in the unknown; Edison failed a thousand times before getting the light bulb right, etc….” The technology is ready when it is ready. In addition, he praised TACF for choosing to explain its decision frankly: “nothing builds credibility like early failures openly admitted.”
Meanwhile, TACF continues to make gains in blight resistance with its traditional American chestnut backcross hybrid breeding program. They have established a genetically diverse, reproducing population of thousands of trees representing hundreds of breeding lines. These trees are planted in TACF’s expansive network of germplasm conservation orchards and regional breeding and backcross orchards. They have substantially increased resistance to both the blight and Phytophthora cinnanomi in these populations. The future inclusion of transgenic and/or gene-edited trees will further increase those gains.
Another Approach
Meantime, the American Chestnut Cooperators Foundation (ACCF), which breeds from persistent pure American chestnut, now has some trees that are nearly 50 years old. The program has bred five generations of pure American chestnuts that show durable blight resistance. Many trees are 60 feet tall or higher; they produce nuts. Vice President Jenny Abla (pers. comm.) reports that they show excellent canker response (swollen and superficial). The picture shows one of their most notable stands, which is in the Jefferson National Forest. Dr. Sniezko is exploring whether this program shows sufficient promise to justify increased support from the USFS.
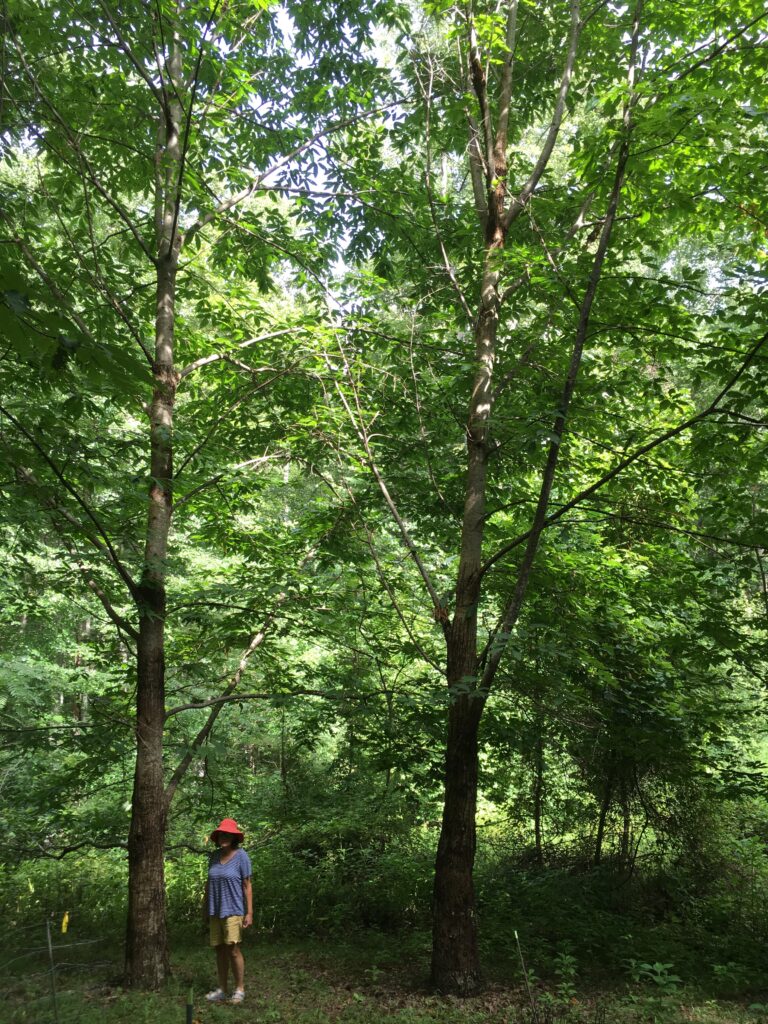
Improving Coordination – will funds follow?
In July 2023, representatives from essentially all the forest tree resistance breeding programs in the U.S. met at Dorena Genetic Resource Center in Oregon to discuss their current successes and how to fast-track all programs. This is the first such meeting since 1982 (Richard Sniezko, pers. comm.). I encourage us all to study the report when it emerges and encourage USFS leadership to support the more unified enterprise.
Status of Efforts to Conserve Other Tree Species
The special issue of New Forests (Vol. 54 Issue 4) included several articles exploring the specifics of breeding elms, ashes, and ʻōhiʻa. These describe difficult challenges … and scientists determined to make progress on overcoming them.
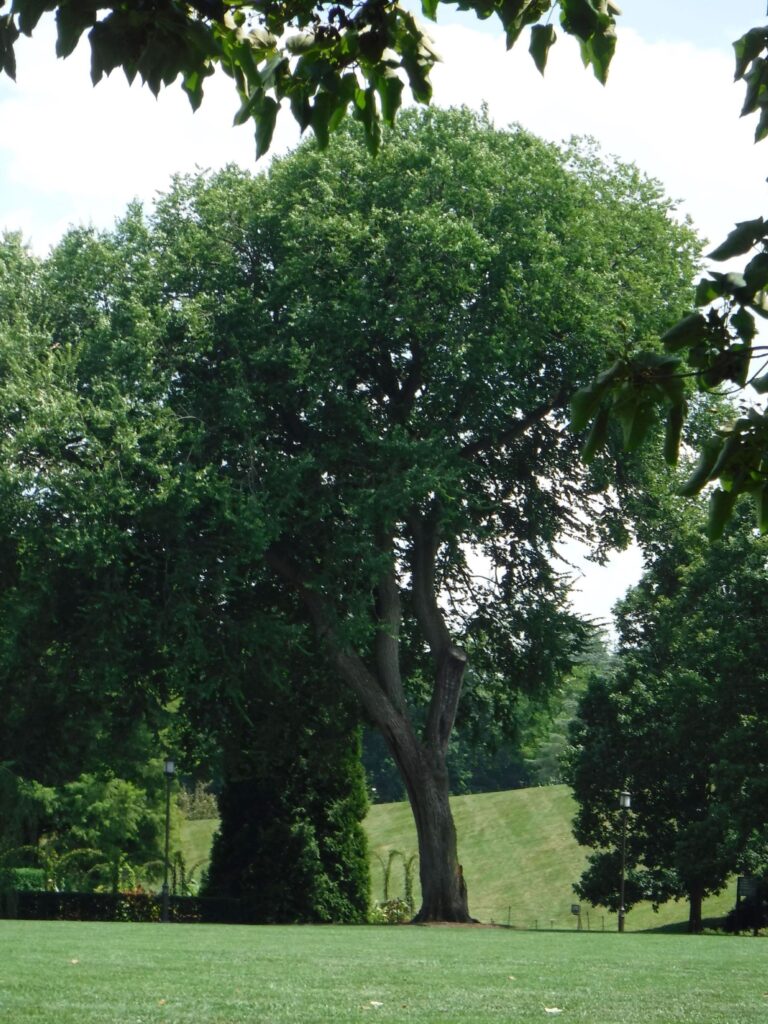
Elms (Ulmus spp.) (see article by Martin et al.)
Let’s not forget that elms were keystone species in Europe and North America until attacked by two epidemics of “Dutch” elm disease during the 20th Century. While hybrid elms are available for urban plantings, many consider them not appropriate for planting in natural forests because these genotypes are not native.
Martin et al. describe a bewildering conglomeration of complexities and possibilities arising from biotic and abiotic factors. Initiation and especially intensity of the disease in a particular tree depend on
- the species or strain of the tree, vectoring beetle, and pathogen;
- timing of the attack; and
- adequacy of water supplies at that time.
Possible targets for manipulation include the pathogen, its beetle vector, and the tree’s response — either in its bark or xylem. Martin et al. suggest that a combination of resistance to the pathogen within the xylem, resistance to beetles’ feeding wounds, and lowering tree clues that attract the beetles could considerably enhance longer-term overall resistance in the field.
However, verifying which approaches produce the best result will be complicated by the trees’ sensitivity to environmental factors such as season and water supply. Apparent resistance might actually be tied to, for example, low water supplies during the spring when the attack occurred.
Restoration strategies, including resistance to pests, must accommodate the diverse ecological conditions in the species’ large range, the rapid evolution of the Ophiostoma pathogens; and other pests and pathogens that attack elms. Nor do scientists know appropriate planting strategies.
Martin et al. believe Dutch elm disease is unlikely to be spread by movement of living elm plants, although other pests could be (and have been).
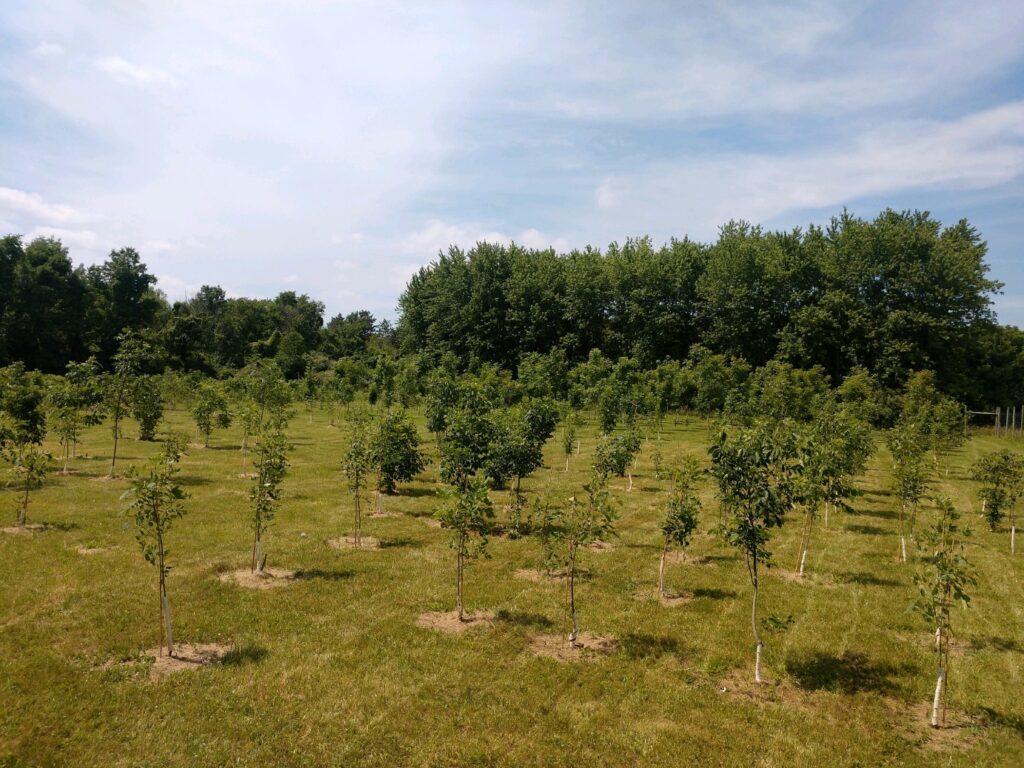
Ashes (Fraxinus spp.)
While a USFS team led by Jennifer Koch link are conducting much of the on-the-ground efforts to breed ash trees resistant to the emerald ash borer (EAB; Agrilus plannipennis), Stanley et al. note that scientists cannot simply cross most North American ash species with the Asian ash, F. mandshurica, because the two groups are sexually incompatible. Scientists have instead focused on trying to enhance the resistance to EAB that is apparently present in a small proportion of ash trees, called “lingering ash.” Scientists funded by USDA Forest Service have already devoted over 14 years to finding such lingering ash to be tested for resistance.
Testing these trees is not simple (see Stanley et al.). But scientists are overcoming some of the obstacles. They have shown that the capability of a few green ash (Fraxinus pennsylvanica) (less than 1%) to defend themselves from EAB attack is genetic. Genes determine the relative abundance of specific metabolites manufactured by the tree; high levels kill more beetle larvae. These trees’ tolerance is not immunity but it might be sufficient to allow the tree to survive and grow. The level of metabolites synthesized by succeeding generations of the tree can probably also be enhanced by breeding.
To restore ash it is necessary to propagate large numbers of clones and to root the resulting embryos. This has been challenging. Merkle et al. describe five years of efforts to develop techniques that allow in vitro propagation to speed up selection and breeding. These techniques will facilitate establishment of numerous groups of propagules with the genetic differences needed to accommodate the large geographic range of several ash trees. For example, the green ash range covers more than half the continental U.S. plus multiple Canadian provinces.
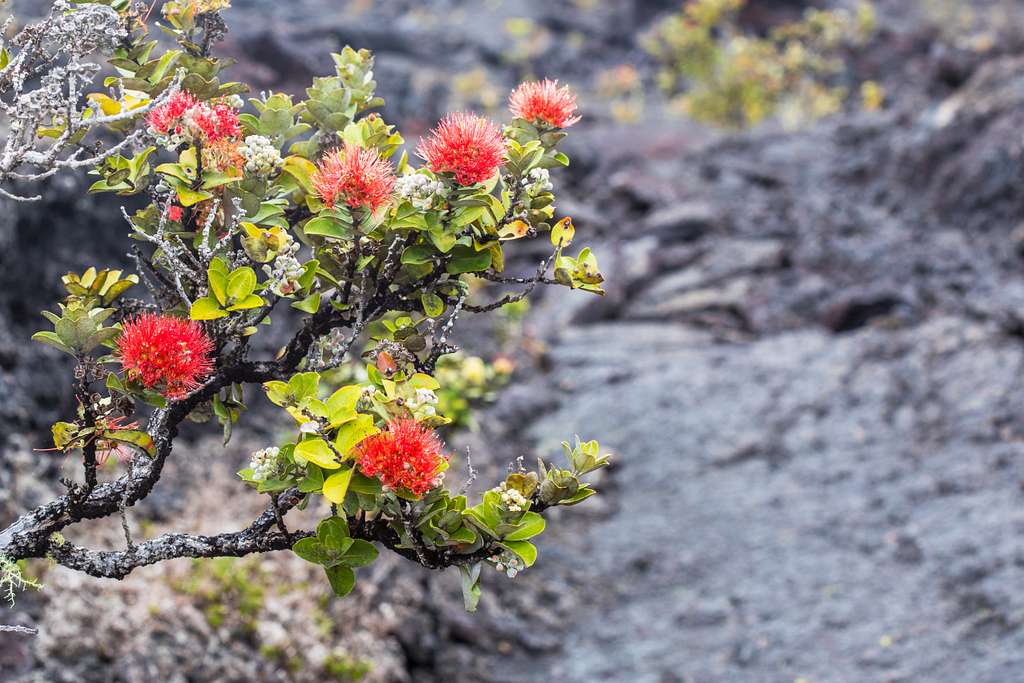
‘Ōhi‘a (Metrosideros polymorpha)
‘Ohi‘a is the most widespread tree species on the Hawaiian Islands. It provides vitally important habitat for conservation of countless taxa of endemic birds, insects, and plants. It is also of great cultural importance for Native Hawaiians.
Luiz et al. review the tree species’ importance, the many threats to native Hawaiian forests, and a coalition’s efforts to counter the most recent – and alarming – threat, rapid ʻōhiʻa death (ROD).
Rapid ʻōhiʻa death is caused by two introduced species of in the genus Ceratocystis. C. lukuohia colonizes the tree’s sapwood and kills the tree quickly. This disease is present on two islands, Hawai`i and Kaua‘i. It has the potential to devastate ‘ohi‘a forests across the state. The other pathogen, C. huliohia, invades the phloem, cambium, and outer xylem, resulting in a well-defined area of necrotic tissue and slower mortality. This disease is on Hawai`i and Kaua‘i, plus Maui and O‘ahu. The two pathogens have different origins. C. lukuohia belongs to a genetic line that is based in Latin America, C. huliohia to a genetic line based in Asia and Australia.
Conservationists formed a coalition and developed a strategy to guide the process of identifying and developing disease resistance in M. polymorpha and, if possible, other Metrosideros species on the Islands. Luiz et al. describe the coalition’s many activities. The challenges are familiar ones:
- obtaining sufficient facilities to screen large numbers of seedlings;
- developing techniques for inoculation, propagation, and speeding up growth of seedlings;
- improving techniques for detecting individual infected and healthy trees across difficult terrain;
- testing trees native to all parts of the tree’s range, which is not large in area, but covers a great variety of elevations and climates); and
- needing to develop trees resistant to both C. lukuohia and C. huliohia.
Luiz et al. reiterate the necessity to manage all threats to healthy ʻōhiʻa stands, for example, by
- curtailing human spead of infected wood, using both quarantines and supportive public education;
- testing repellants to reduce beetle attack.
- reducing injuries to trees by fencing forests and removing feral ungulates. link to website?
SOURCES
Buggs, R.J.A. 2020. Changing perceptions of tree resistance research. Plants, People, Planet. 2020;2:2–4. https://doi.org/10.1002/ppp3.10089
Coleman, T.W., A.D. Graves, B.W. Oblinger, R.W. Flowers, J.J. Jacobs, B.D. Moltzan, S.S. Stephens, R.J. Rabaglia. 2023. Evaluating a decade (2011–2020) of integrated forest pest management in the United States. Journal of Integrated Pest Management. (2023) 14(1): 23; 1–17
Dudley, N.; Jones, T.; Gerber, K.; Ross-Davis, A.L.; Sniezko, R.A.; Cannon, P.; Dobbs, J. 2020. Establishment of a Genetically Diverse, Disease-Resistant Acacia koa A. Gray Seed Orchard in Kokee, Kauai: Early Growth, Form, and Survival. Forests 2020, 11, 1276 https://doi.org/10.3390/f11121276
Jacobs, D.F., R. Kasten Dumroese, A.N. Brennan, F.T. Campbell, A.O. Conrad, J.A. Delborne, et al. 2023. Reintroduction of at-risk forest tree species using biotech depends on regulatory policy, informed
by science and with public support. New Forests (2023) 54:587–604
Luiz, B.C., C.P. Giardina, L.M. Keith, D.F. Jacobs, R.A. Sniezko, M.A. Hughes, J.B. Friday, P. Cannon, R. Hauff, K. Francisco, M.M. Chau, N. Dudley, A. Yeh, G. Asner, R.E. Martin, R. Perroy, B.J. Tucker, A. Evangelista, V. Fernandez, C. Martins-Keli.iho.omalu, K. Santos, R. Ohara. 2023. A framework for establishing a rapid ‘Ohi‘a death resistance program. New Forests https://doi.org/10.1007/s11056-021-09896-5
Martín, J.A., J. Domínguez, A. Solla, C.M. Brasier, J.F. Webber, A. Santini, C. Martínez-Arias, L. Bernier, L. Gil1. 2023. Complexities underlying the breeding and deployment of Dutch elm disease resistant elms. New Forests https://doi.org/10.1007/s11056-021-09865-y
Merkle, S.A., J.L. Koch, A.R. Tull, J.E. Dassow, D.W. Carey, B.F. Barnes, M.W.M. Richins, P.M. Montello, K.R. Eidle, L.T. House, D.A. Herms and K.J.K. Gandhi. 2023. Application of somatic embryogenesis for development of emerald ash borer-resistant white ash and green ash varietals. New Forests https://doi.org/10.1007/s11056-022-09903-2
Nelson, C.D. 2023. Tree breeding, a necessary complement to genetic engineering. New Forests
Pike, C.C., J. Koch, C.D. Nelson. 2021. Breeding for Resistance to Tree Pests: Successes, Challenges, and a Guide to the Future. Journal of Forestry, Volume 119, Issue 1, January 2021, Pages 96–105, https://doi.org/10.1093/jofore/fvaa049
Sniezko, R.A., J. Koch, J-J. Liu and J. Romero-Severson. 2023. Will Genomic Info Facilitate Forest Tree Breeding for Disease and Pest Resistance? Forests 2023, 14, 2382.
Sniezko, R.A. and C.D. Nelson. 2022. Chapter 10, Resistance breeding against tree pathogens. In Asiegbu and Kovalchuk, editors. Forest Microbiology Volume 2: Forest Tree Health; 1st Edition. Elsevier
Stanley, R.K., Carey, D.W., Mason, M.E., Doran, A., Wolf, J., Otoo, K.O., Poland, T.M., Koch, J.L., Jones, A.D. and Romero-Severson, J. 2023. Emerald ash borer (Agrilus planipennis) infestation bioassays and metabolic profiles of green ash (Fraxinus pennsylvanica) provide evidence for an induced host defensive response to larval infestation. Front. For. Glob. Change 6:1166421. doi: 10.3389/ffgc.2023.1166421
Posted by Faith Campbell
We welcome comments that supplement or correct factual information, suggest new approaches, or promote thoughtful consideration. We post comments that disagree with us — but not those we judge to be not civil or inflammatory.
For a detailed discussion of the policies and practices that have allowed these pests to enter and spread – and that do not promote effective restoration strategies – review the Fading Forests report at http://treeimprovement.utk.edu/FadingForests.htm
or